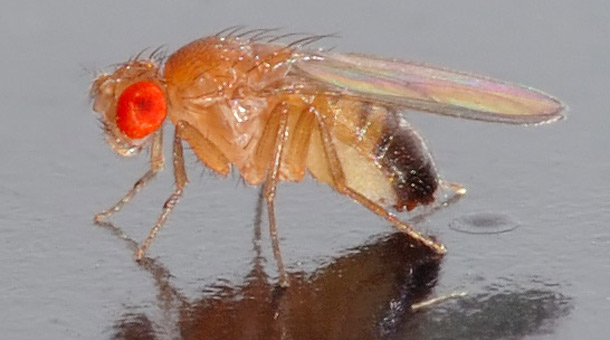
Speciation is the evolutionary process by which new biological species arise. Speciation can occur from a variety of different causes and are classified in various forms (e.g. allopatric, sympatric, polyploidization, etc.). Scientists have observed numerous examples of speciation in the laboratory and in nature, however, evolution has produced far more species than an observer would consider necessary. For example, there are well over 350,000 described species of beetles. Great examples of observed speciation come from the observations of island biogeography and the process of adaptive radiation, both explained in an earlier section. The examples shown below provide strong evidence for common descent and are only a small fraction of the instances observed.
Blackcap
Drosophila Melanogaster
They then cultured two strains that chose opposite habitats. One of the strains emerged early, immediately flying upward in the dark attracted to the acetaldehyde. The other strain emerged late and immediately flew downward, attracted to light and ethanol. Pupae from the two strains were then placed together in the maze and allowed to mate at the food site. They then were collected. A selective penalty was imposed on the female flies that switched habitats. This entailed that none of their gametes would pass on to the next generation. After 25 generations of this mating test, it showed reproductive isolation between the two strains. They repeated the experiment again without creating the penalty against habitat switching and the result was the same; reproductive isolation was produced
Hawthorn Fly
London Underground Mosquito
This mosquito, although first discovered in the London Underground system, has been found in underground systems around the world. It is suggested that it may have adapted to human-made underground systems since the last century from local above-ground Culex pipiens, although more recent evidence suggests that it is a southern mosquito variety related to Culex pipiens that has adapted to the warm underground spaces of northern cities.
The species have very different behaviours, are extremely difficult to mate, and with different allele frequency, consistent with genetic drift during a founder event. More specifically, this mosquito, Culex pipiens molestus, breeds all-year round, is cold intolerant, and bites rats, mice, and humans, in contrast to the above ground species Culex pipiens that is cold tolerant, hibernates in the winter, and bites only birds. When the two varieties were cross-bred the eggs were infertile suggesting reproductive isolation.
The fundamental results still stands: the genetic data indicate that the molestus form in the London Underground mosquito appeared to have a common ancestry, rather than the population at each station being related to the nearest above-ground population (i.e. the pipiens form). Byrne and Nichols’ working hypothesis was that adaptation to the underground environment had occurred locally in London once only.
These widely separated populations are distinguished by very minor genetic differences, which suggest that the molestus form developed: a single mtDNA difference shared among the underground populations of ten Russian cities; a single fixed microsatellite difference in populations spanning Europe, Japan, Australia, the middle East and Atlantic islands.
Madeira House Mouse
Mollies
Polar Bear
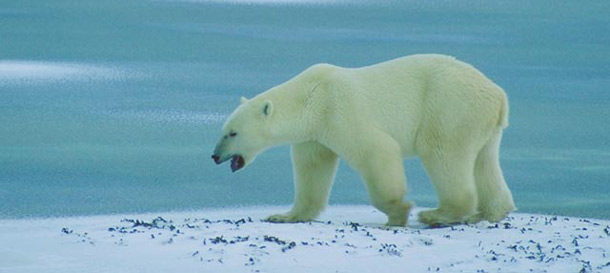
A specific example of large-scale evolution is the polar bear (Ursus maritimus). The polar bear is related to the brown bear (Ursus arctos) but they can still interbreed and produce fertile offspring. However, it has acquired significant physiological differences from the brown bear. These differences allow the polar bear to comfortably survive in conditions that the brown bear could not including the ability to swim sixty miles or more at a time in freezing waters, to blend in with the snow, and to stay warm in the arctic environment. Additionally, the elongation of the neck makes it easier to keep their heads above water while swimming and the oversized webbed feet that act as paddles when swimming. The polar bear has also evolved small papillae and vacuole-like suction cups on the soles to make them less likely to slip on the ice; feet covered with heavy matting to protect the bottoms from intense cold and provide traction; smaller ears to reduce the loss of heat; eyelids that act like sunglasses; accommodations for their all-meat diet; a large stomach capacity to enable opportunistic feeding; and the ability to fast for up to nine months while recycling their urea.
Thale Cress
To test this, Bomblies crossed 280 genetically different strains of Arabidopsis in 861 distinct ways and found that 2 per cent of the resulting hybrids were necrotic. Along with allocating the same indicators, the 20 plants also shared a comparable collection of genetic activity in a group of 1,080 genes. In almost all of the cases, Bomblies discovered that only two genes were required to cause the autoimmune response. Bomblies looked at one hybrid in detail and found that one of the two genes belonged to the NB-LRR class, a common group of disease resistance genes involved in recognizing new infections. When Bomblies removed the problematic gene, the hybrids developed normally.
Over successive generations, these incompatibilities could create divisions between different plant strains, reducing their chances of successful mating and turning distinct strains into separate species.
Salsify
T. dubius and T. pratensis mated in Europe but were never able to hybridize. A study published in March 2011 found that when these two plants were introduced to North America in the 1920s, they mated and doubled the number of chromosomes in there hybrid Tragopogon miscellus allowing for a “reset” of its genes, which in turn, allows for greater genetic variation. Professor Doug Soltis of the University of Florida said, “We caught evolution in the act…New and diverse patterns of gene expression may allow the new species to rapidly adapt in new environments”. This observable event of speciation through hybridization further advances the evidence for the common descent of organisms and the time frame in which the new species arose in its new environment. The hybridizations have been reproduced artificially in laboratories from 2004 to present day.
Welsh Groundsel
York Grounsel
It resulted from a backcrossing of the F1 hybrid of its parents to S. vulgaris. S. vulgaris is native to Britain, while S. squalidus was introduced from Sicily in the early 18th century; therefore, S. eboracensis has speciated from those two species within the last 300 years.
Other hybrids descended from the same two parents are known. Some are infertile, such as S. x baxteri. Other fertile hybrids are also known, including S. vulgaris var. hibernicus, now common in Britain, and the allohexaploid S. cambrensis, which according to molecular evidence probably originated independently at least three times in different locations. Morphological and genetic evidence support the status of S. eboracensis as separate from other known hybrids.
James K. Liebherr and Joseph V. McHugh in Resh, V. H. & R. T. Cardé (Editors) 2003. Encyclopedia of Insects. Academic Press.
Bearhop, S.; Fiedler, W; Furness, RW; Votier, SC; Waldron, S; Newton, J; Bowen, GJ; Berthold, P et al. (2005). “Assortative mating as a mechanism for rapid evolution of a migratory divide”. Science 310 (5747): 502–504. Bibcode 2005Sci…310..502B. doi:10.1126/science.1115661. PMID 16239479. Supporting Online Material
Ed Yong (December 3, 2009). “British birdfeeders split blackcaps into two genetically distinct groups : Not Exactly Rocket Science”. ScienceBlogs. Retrieved 2010-05-21.
William R. Rice, George W. Salt (1990). “The Evolution of Reproductive Isolation as a Correlated Character Under Sympatric Conditions: Experimental Evidence”. Evolution, Society for the Study of Evolution 44.
“he Evolution of Reproductive Isolation as a Correlated Character Under Sympatric Conditions: Experimental Evidence”. William R. Rice, George W. Salt. Retrieved 2010-05-23.
“Observed Instances of Speciation, 5.3.5 Sympatric Speciation in Drosophila melanogaster”. Joseph Boxhorn. Retrieved 2010-05-23.
Feder JL, Roethele JB, Filchak K, Niedbalski J, Romero-Severson J (1 March 2003). “Evidence for inversion polymorphism related to sympatric host race formation in the apple maggot fly, Rhagoletis pomonella”. Genetics 163 (3): 939–53. PMC 1462491. PMID 12663534.
Berlocher SH, Bush GL (1982). “An electrophoretic analysis of Rhagoletis (Diptera: Tephritidae) phylogeny”. Systematic Zoology 31 (2): 136–55. doi:10.2307/2413033. JSTOR 2413033.
Berlocher SH, Feder JL (2002). “Sympatric speciation in phytophagous insects: moving beyond controversy?”. Annu Rev Entomol. 47: 773–815. doi:10.1146/annurev.ento.47.091201.145312. PMID 11729091.
Bush GL (1969). “Sympatric host race formation and speciation in frugivorous flies of the genus Rhagoletis (Diptera: Tephritidae)”. Evolution 23 (2): 237–51. doi:10.2307/2406788. JSTOR 2406788.
Prokopy RJ, Diehl SR, Cooley SS (1988). “Behavioral evidence for host races in Rhagoletis pomonella flies”. Oecologia 76 (1): 138–47. JSTOR 4218647.
Feder JL, Roethele JB, Wlazlo B, Berlocher SH (1997). “Selective maintenance of allozyme differences among sympatric host races of the apple maggot fly”. Proc Natl Acad Sci USA. 94 (21): 11417–21. Bibcode 1997PNAS…9411417F. doi:10.1073/pnas.94.21.11417. PMC 23485. PMID 11038585.
“London underground source of new insect forms”. The Times. 1998-08-26.
Fonseca, D. M.; Keyghobadi, N; Malcolm, CA; Mehmet, C; Schaffner, F; Mogi, M; Fleischer, RC; Wilkerson, RC (2004). “Emerging vectors in the Culex pipiens complex”. Science 303 (5663): 1535–8. Bibcode 2004Sci…303.1535F. doi:10.1126/science.1094247. PMID 15001783.
Alan Burdick (2001). “Insect From the Underground — London, England Underground home to different species of mosquitos”. Natural History.
Byrne K, Nichols RA (1999). “Culex pipiens in London Underground tunnels: differentiation between surface and subterranean populations”. Heredity 82 (1): 7–15. doi:10.1038/sj.hdy.6884120. PMID 10200079.
Vinogradova EB and Shaikevich EV (2007). “Morphometric, physiological and molecular characteristics of underground populations of the urban mosquito Culex pipiens Linnaeus f. molestus Forskål (Diptera: Culicidae) from several areas of Russia”. European Mosquito Bulletin 22: 17–24.
Britton-Davidian, Janice; Catalan, Josette; Da Graça Ramalhinho, Maria; Ganem, Guila; Auffray, Jean-Christophe; Capela, Ruben; Biscoito, Manuel; Searle, Jeremy B. et al. (2000). “Rapid chromosomal evolution in island mice”. Nature 403 (6766): 158. Bibcode 2000Natur.403..158B. doi:10.1038/35003116. PMID 10646592.
Tobler, Micheal (2009). Does a predatory insect contribute to the divergence between cave- and surface-adapted fish populations? Biology Letters doi:10.1098/rsbl.2009.0272
“Giant insect splits cavefish into distinct populations”. Ed Yong. Retrieved 2010-05-22.
Bomblies, Kirsten; Lempe, Janne; Epple, Petra; Warthmann, Norman; Lanz, Christa; Dangl, Jeffery L.; Weigel, Detlef (2007). “Autoimmune Response as a Mechanism for a Dobzhansky-Muller-Type Incompatibility Syndrome in Plants”. PLoS Biol 5 (9): e236. doi:10.1371/journal.pbio.0050236. PMC 1964774. PMID 17803357.
“New plant species arise from conflicts between immune system genes”. Ed Yong. Retrieved 2010-05-22.
Adaptive Traits of the Polar Bear (Ursus Maritimus). Scienceray.com (2008-08-13). Retrieved on 2011-12-06.
Polar Bear Evolution. Polarbearsinternational.org (2011-12-01). Retrieved on 2011-12-06.
Ron Rayborne Accepts Hovind’s Challenge
Karpechenko, G.D., Polyploid hybrids of Raphanus sativus X Brassica oleracea L., Bull. Appl. Bot. 17:305–408 (1927).
Terasawa, Y. Crossing between Brassico-raphanus and B. chinensis and Raphanus sativus. Japanese Journal of Genetics. 8(4): 229–230 (1933).
William Kirkwood Purves, David E. Sadava, Gordon H. Orians, and H. Craig Heller (2006). Life, the science of biology (7 ed.). Sinaur Associates, Inc.. p. 487. ISBN 0-7167-9856-5.
Pam Soltis (2011-03-17). “UF researcher: Flowering plant study ‘catches evolution in the act'”. EurekAlert, American Association for the Advancement of Science. Retrieved 2011-03-28.
Buggs, Richard J.A.; Zhang, Linjing; Miles, Nicholas; Tate, Jennifer A.; Gao, Lu; Wei, Wu; Schnable, Patrick S.; Barbazuk, W. Brad et al. (2011). “Transcriptomic Shock Generates Evolutionary Novelty in a Newly Formed, Natural Allopolyploid Plant”. Current Biology 21 (7): 551–6. doi:10.1016/j.cub.2011.02.016. PMID 21419627.
Andrew J. Lowe, Richard J. Abbott (1996). “Origins of the New Allopolyploid Species Senecio camrensis (asteracea) and its Relationship to the Canary Islands Endemic Senecio tenerifae”. American Journal of Botany 83 (10): 1365–1372. doi:10.2307/2446125. JSTOR 2446125.
Jerry A. Coyne (2009). Why Evolution is True. Penguin Group. pp. 187 – 189. ISBN 978-0-670-02053-9.
Missouri Botanical Garden. “TROPICOS Web display Senecio vulgaris L.”. Nomenclatural and Specimen Data Base. Missouri State Library. Retrieved 2008-02-01.