
Natural selection will only cause evolution if there is enough genetic variation in a population. Before the discovery of Mendelian genetics, one common hypothesis was blending inheritance. But with blending inheritance, genetic variance would be rapidly lost, making evolution by natural selection implausible. The Hardy-Weinberg principle provides the solution to how variation is maintained in a population with Mendelian inheritance. The frequencies of alleles (variations in a gene) will remain constant in the absence of selection, mutation, migration and genetic drift.
Variation comes from mutations in genetic material, reshuffling of genes through sexual reproduction and migration between populations (gene flow). Despite the constant introduction of new variation through mutation and gene flow, most of the genome of a species is identical in all individuals of that species. However, even relatively small differences in genotype can lead to dramatic differences in phenotype: for example, chimpanzees and humans differ in only about 5% of their genomes.
Among Individuals Within a Population
Genetic variation can also be identified by examining variation at the level of enzymes using the process of protein electrophoresis. Polymorphic genes have more than one allele at each locus. Half of the genes that code for enzymes in insects and plants may be polymorphic, whereas polymorphisms are less common in vertebrates.
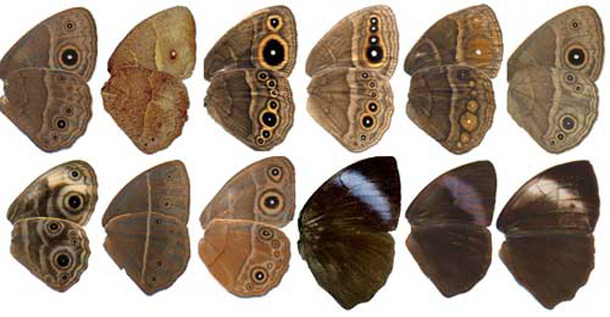
Ultimately, genetic variation is caused by variation in the order of bases in the nucleotides in genes. New technology now allows scientists to directly sequence DNA which has identified even more genetic variation than was previously detected by protein electrophoresis. Examination of DNA has shown genetic variation in both coding regions and in the non-coding intron region of genes.
Genetic variation will result in phenotypic variation if variation in the order of nucleotides in the DNA sequence results in a difference in the order of amino acids in proteins coded by that DNA sequence, and if the resultant differences in amino acid sequence influence the shape, and thus the function of the enzyme.
Mutation
Mutations can involve large sections of a chromosome becoming duplicated (usually by genetic recombination), which can introduce extra copies of a gene into a genome. Extra copies of genes are a major source of the raw material needed for new genes to evolve. This is important because most new genes evolve within gene families from pre-existing genes that share common ancestors. For example, the human eye uses four genes to make structures that sense light: three for colour vision and one for night vision; all four are descended from a single ancestral gene.
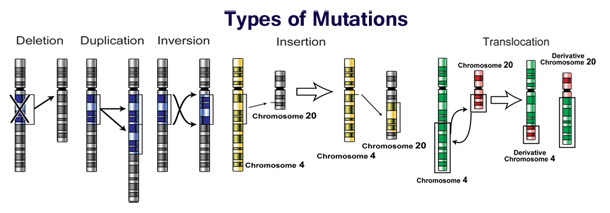
New genes can be generated from an ancestral gene when a duplicate copy mutates and acquires a new function. This process is easier once a gene has been duplicated because it increases the redundancy of the system; one gene in the pair can acquire a new function while the other copy continues to perform its original function. Other types of mutations can even generate entirely new genes from previously noncoding DNA.
The generation of new genes can also involve small parts of several genes being duplicated, with these fragments then recombining to form new combinations with new functions. When new genes are assembled from shuffling pre-existing parts, domains act as modules with simple independent functions, which can be mixed together to produce new combinations with new and complex functions. For example, polyketide synthases are large enzymes that make antibiotics; they contain up to one hundred independent domains that each catalyze one step in the overall process, like a step in an assembly line.
Sturm RA, Frudakis TN (2004). “Eye colour: portals into pigmentation genes and ancestry”. Trends Genet. 20 (8): 327–32. doi:10.1016/j.tig.2004.06.010. PMID 15262401.
Pearson H (2006). “Genetics: what is a gene?”. Nature 441 (7092): 398–401. Bibcode 2006Natur.441..398P. doi:10.1038/441398a. PMID 16724031.
Visscher PM, Hill WG, Wray NR (2008). “Heritability in the genomics era—concepts and misconceptions”. Nat. Rev. Genet. 9 (4): 255–66. doi:10.1038/nrg2322. PMID 18319743.
Oetting WS, Brilliant MH, King RA (1996). “The clinical spectrum of albinism in humans”. Molecular medicine today 2 (8): 330–5. doi:10.1016/1357-4310(96)81798-9. PMID 8796918.
Futuyma, Douglas J. (2005). Evolution. Sunderland, Massachusetts: Sinauer Associates, Inc. ISBN 0-87893-187-2.
Phillips PC (2008). “Epistasis—the essential role of gene interactions in the structure and evolution of genetic systems”. Nat. Rev. Genet. 9 (11): 855–67. doi:10.1038/nrg2452. PMC 2689140. PMID 18852697.
Wu R, Lin M (2006). “Functional mapping – how to map and study the genetic architecture of dynamic complex traits”. Nat. Rev. Genet. 7 (3): 229–37. doi:10.1038/nrg1804. PMID 16485021.
Jablonka, E.; Raz, G. (2009). “Transgenerational epigenetic inheritance: Prevalence, mechanisms and implications for the study of heredity and evolution.”. The Quarterly Review of Biology 84 (2): 131–176. doi:10.1086/598822. PMID 19606595.
Bossdorf, O.; Arcuri, D.; Richards, C. L.; Pigliucci, M. (2010). “Experimental alteration of DNA methylation affects the phenotypic plasticity of ecologically relevant traits in Arabidopsis thaliana”. Evolutionary Ecology 24 (3): 541–553. doi:10.1007/s10682-010-9372-7.
Jablonka, E.; Lamb, M. (2005). Evolution in four dimensions: Genetic, epigenetic, behavioural and symbolic. MIT Press. ISBN 0-262-10107-6.
Jablonka, E.; Lamb, M. J. (2002). “The changing concept of epigenetics”. Annals of the New York Academy of Sciences 981 (1): 82–96. Bibcode 2002NYASA.981…82J. doi:10.1111/j.1749-6632.2002.tb04913.x. PMID 12547675.
Laland, K. N.; Sterelny, K. (2006). “Perspective: Seven reasons (not) to neglect niche construction”. Evolution 60 (8): 1751–1762. doi:10.1111/j.0014-3820.2006.tb00520.x
Bijma, P.; Wade, M. J. (2008). “The joint effects of kin, multilevel selection and indirect genetic effects on response to genetic selection”. Journal of Evolutionary Biology 21 (5): 1175–1188. doi:10.1111/j.1420-9101.2008.01550.x. PMID 18547354.
Vrba, E. S.; Gould, S. J. (1986). “The hierarchical expansion of sorting and selection: Sorting and selection cannot be equated”. Paleobiology 12 (2): 217–228.
Henig, Robin Marantz (2001). The Monk in the Garden : The Lost and Found Genius of Gregor Mendel, the Father of Genetics. Houghton Mifflin. ISBN 0-395-97765-7. “The article, written by an obscure Moravian monk named Gregor Mendel”
Carlson, Neil and et al. “Psychology the Science of Bahaviour”, p. 206. Pearson Canada, United States of America. ISBN 978-0-205-64524-4.
Mayr E. 1982. The growth of biological thought: diversity, evolution & inheritance. Harvard, Cambs. p567 et seq.
Gould S.J. Ontogeny and phylogeny. Harvard 1977. p221-2